The radiation is produced
by a beam of 6.04 GeV electrons circulating in a storage
ring. The electrons radiate synchrotron radiation in
the bending magnets of the ring lattice and in magnetic
insertion devices wigglers and undulators
that shake the beam.
However, the new Graal experiment at the ESRF generates
radiation in another way to probe nuclear and nucleon
structure.
Graal has been realized
by a French-Italian-Russian collaboration with primary
financial support from INFN in Italy and IN2P3 in France
through several Italian laboratories and universities
(Laboratori Nazionali di Frascati, Laboratori Nazionali
del Sud, and the universities of Catania, Genova, Roma
II and Torino), two French Institutes (Institut des
Sciences Nucleaires and Institut de Physique Nucleaire)
and the Institute for Nuclear Research of the Russian
Academy of Science.

Undulators are normally composed of physical magnets,
but Graal uses a micro-undulator in the ESRF straight
section D7,which is a beam of ultraviolet laser light
moving against the electron beam. The electrons scatter
the laser photons, transferring energy to them and producing
narrowly collimated gamma rays.
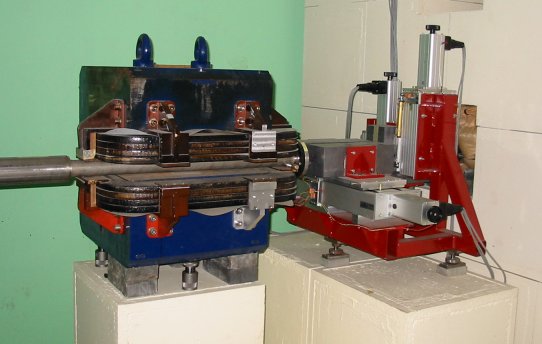
At the ESRF, a laser beam in the near-ultraviolet produces
gamma rays with a maximum energy of 1.47 GeV, a maximum
linear polarization of 98% and an intensity of a few
millions of photons per second. In this way, Graal extends
the ESRF resolving power to nuclear and that of nucleon
structure down to a spatial resolution of 0.2 fm (0.2
x 10-13 cm).
The first advantage of this technique over normal electron
bremsstrahlung synchrotron radiation is the almost flat
energy spectrum. Polarization is the second advantage:
photons scattered in the electron direction maintain
their polarization. Therefore, at the higher end of
the spectrum, the polarization is very close to that
of the laser light. Rotating or changing the polarization
of the gamma rays is easily accomplished by rotating
or changing the polarization of the laser light.

A gamma beam of a useful intensity for studying photonuclear
reactions was first produced at Frascati using the Adone
storage ring. After this success, several more such
beams were produced.
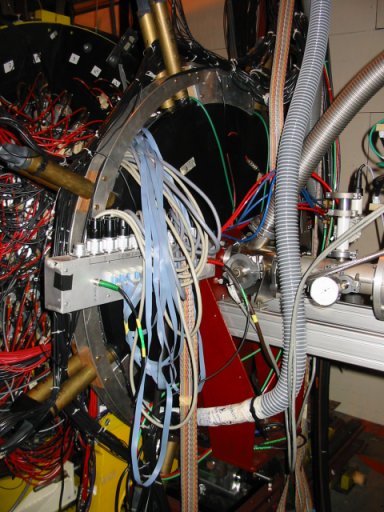
A beam with a maximum
energy of 2.4 GeV is now under construction at Spring8
in Japan.
Graal's main goal is the study of photonuclear reactions
in the intermediate energy region, where the nucleons
cannot be treated as elementary particles and their
internal degrees of freedom cannot be ignored, but away
from the asymptotic freedom of quarks and gluons. In
this region, many excited baryon states are clearly
visible and many others await careful exploration
As highlighted by Feynman's quotation, photons are an
interesting probe of hadronic structure because the
interaction is given by the product of the electromagnetic
vector potential and the hadronic current. The former
is well known from quantum electrodynamics, and the
relative weakness of electromagnetic coupling makes
second-order effects small, thus models of photonuclear
reactions are possible. Moreover, the possibility of
using linearly and circularly polarized gamma rays makes
several single- and double-polarization observables
experimentally accessible, providing strong constraints
on theoretical models. A linearly polarized photon beam
introduces a fixed direction for the electric field,
so that the reaction yield is no longer cylindrically
symmetric with respect to the beam direction.
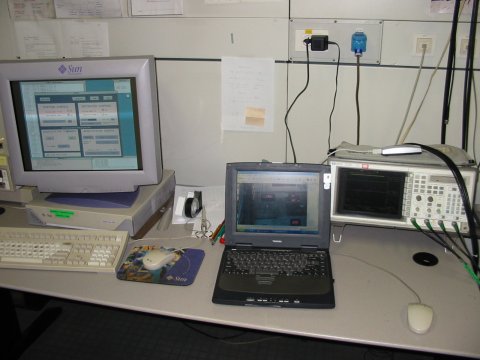
In a circularly polarized beam, the photons have well
defined helicity and their spins are aligned parallel
or antiparallel to their momentum. Parity conservation
in photoreactions dictates the overall form of the interaction.
The asymmetry in the weak decay of the (strange) lambda
provides information on lambda polarization, so it is
possible to measure the correlation between the gamma
and lambda polarizations in the photoproduction of strange
particles.
Ultraviolet
photon beam
In Graal, an argon-ion laser provides a beam of ultraviolet
photons. A three-lens zoom focuses them at the center
of the laserelectron interaction region. Two precisely
adjustable mirrors align the laser light with the electron
beam 35 m away to within 3 µrad. A retardation
plate rotates the plane of linear polarization of the
beam. The ultraviolet enters the storage ring vacuum
system through a quartz window and is subsequently reflected
through 90° by a beryllium mirror coated with aluminium.
This mirror lines up the laser beam with the electron
beam. (Beryllium minimizes the absorption of back scattered
gamma rays that travel in the opposite direction to
the ultraviolet.)

Electrons that have transferred part of their energy
to a photon, move with the electron bunch along the
straight section but, owing to their lower energy, veer
away in the next dipole and become separated from the
un scattered electrons by a few centimeters. Measuring
the distance between a scattered electron and the electron
beam is a measure of its energy loss and therefore of
the gamma energy.
The detection of the scattered electron and the measurement
of its precise position are done with the tagging detector,
which comprises plastic scintillators and silicon microstrips.
The microstrips give the position of the electron and
the scintillators give its precise timing. The electron
timing correlates an event in the hadronic detector
with the corresponding electron, thus providing the
energy of the gamma ray that produced it. It also provides
a precise starting signal for measuring the time of
flight (TOF) of photoproduced particles. The jitter
of the TOF start pulse, provided by the scintillators,
is reduced to 120 ps, effectively synchronizing this
pulse with the phase of the accelerating radiofrequency
of the ring. This is possible because the electrons
travel in short bunches separated by 2.8 ns.
The Graal hadronic detector covers the entire solid
angle except for two small entry and exit holes along
the beam axis. The detector is made of three parts.
In the central part, between 25° and 155°, the
emerging particles pass through two cylindrical wire
chambers and a barrel of 32 thin plastic scintillators,
then enter a calorimeter made of 480 BGO crystals, each
24 cm long, and arranged, like an orange, in 32 sectors
of 15 crystals each.

Particles emitted at angles of less than 25° go
through two plane wire chambers and three plastic scintillator
walls. The first two thin walls are used to measure
the specific ionization of the particles. Then a thick
wall, with alternating layers of plastic scintillator
and lead, measures the total charged particle energy
and detects neutrons and gamma rays. All three walls
provide a measurement of the position and time of flight
of the particles.
Particles emitted backwards encounter two plastic scintillator
discs separated by lead. Each disc has a small central
hole for the passage of the beam and is viewed by 12
photomultipliers to reconstruct the position and timing
of a particle. The responses of the two discs allow
charged particles and gamma rays to be differentiated.
The main features of the Graal detector are high efficiency,
good energy resolution for gamma-ray detection and complete
angular coverage. The detector is well suited to events
producing several photons, like the photoproduction
of neutral pions and etas, and the identification of
the various eta decay channels. The first results to
emerge are extensive measurements of the beam polarization
asymmetries for the photoproduction of positive and
neutral pions and etas. The two-photon and three-neutral-pion
(giving six photons) decay channels of the eta have
been detected simultaneously.
Polarization asymmetries, derived experimentally from
the ratio of successive measurements with the same apparatus,
are immune to otherwise common systematic experimental
errors, such as the knowledge of the solid angle, the
efficiency of the apparatus, the measurement of the
dose and the size of the target. From a theoretical
point of view, polarization asymmetries are given by
the interference of different amplitudes and are therefore
more sensitive to small, hitherto unobserved, contributions
if b is much less than a, then ab is more sensitive
to b than is a2 + b2.
Event
discrimination
Another advantage of full solid-angle apparatus, with
a high overall efficiency for the detection of gamma
rays, is its ability to discriminate rare events, where
only one or a few photons are produced, from the more
frequent events containing many gammas.
One example is Compton scattering, which is about 50
times as rare as neutral pion photoproduction and can
be difficult to single out using only kinematics. However,
Compton scattering has only one photon in the final
state, while neutral pion photoproduction has two. Another
example is the rare decay of the eta into a neutral
pion, which has four photons in the final state compared
with the frequent decay into three neutral pions, which
has six.

Graal is now in full operation. It can collect data
for more than six months per year a large fraction
of the time that the ESRF ring is available to the experimenters.
A 10 mK dilution refrigerator and a 16 T magnet are
now being delivered for the construction of a polarized
target. With polarized targets, double-polarization
experiments will be possible in all channels.
|